Carn Owen: how a modest
Mid-Wales hill records drastic past climate change - the Hirnantian
Glaciation
BACK
TO
WEATHER-BLOG
MENU
New!
Fine
Art
Prints
&
digital
images
for
sale-
Welsh Weather
& Dyfi Valley landscapes Slide-Library - Click HERE
Note: a version of this
post for people less familiar with geology can be found HERE.
"The
climate
has
always
been
changing",
or
words
to
that effect. How many
times have you read that little gem of wisdom in online discussions or
Tabloid op-eds? It usually means that the author of the comment has run
out of other arguments with which to attempt to rubbish climate
science.
So what's the answer?
Sure it has! The rocks in my local patch - Mid-Wales - span
a
time,
over
440
million
years
ago,
when
a remarkable period of climate
change occurred - and they record its passing. However, before anyone
gets excited, I should add an
afternote. The change was accompanied by the second biggest
mass-extinction in the Phanerozoic (last 542 million years) fossil
record. Mr Tabloid Writer might like to pause to reflect upon that for
a
moment. In the meantime, I'll set the scene.
The
rolling hills of the Cambrian Mountains, around the Nant-y-moch
hydro-electric reservoir, are the old stamping-ground for all former
students from the Geology Department at
Aberystwyth University, myself included. The quarried ridge of Carn
Owen - circled in the map below -
is where countless Aber geology undergrads first learned the art of
geological mapping. It lends itself admirably to the purpose - there's
lots to see in less than a square kilometre....
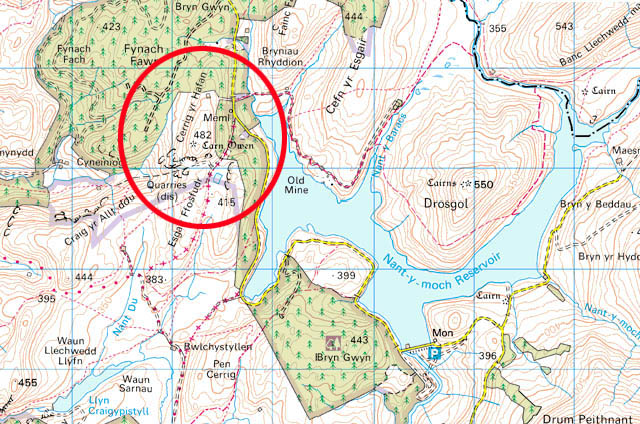
Image credit: Ordnance Survey, from
the excellent Ordnance Survey Get-a-map
service.
Here's a quick look at the Phanerozoic timescale - the past 542 million
years - for context:

I've abbreviated the Period names for the
sake of space. The relevant ones here are:
ORD = Ordovician Period, 488.3-443.7 and SIL =
Silurian Period, 443.7-416 million years ago.
With that in mind, let's have a look
around....
The first thing that catches the eye on arrival at Carn Owen is the big
main quarry, with faces tens of metres high, that was last worked in
the
1950s when the Nant-y-moch dam
was under construction....
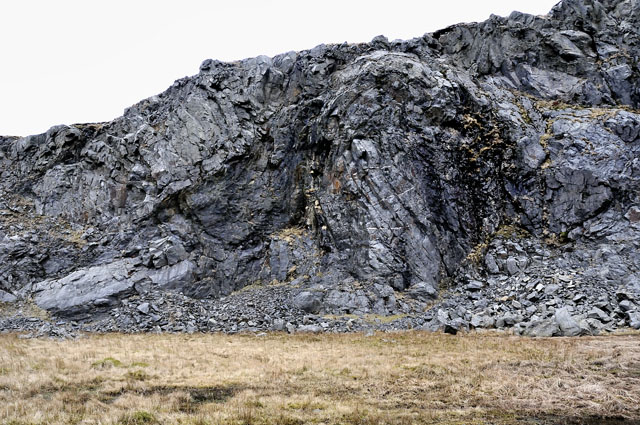
The quarrymen who worked in
this windswept spot exploited hard sandstones that belong to the
Drosgol Formation, of late Ordovician
age (see reference 4 for full local geology). Around
to the left, a short steep ascent
over grass,
mosses and cropped bilberry leads to the summit, with its ancient
ring-cairn,
made of sandstone blocks:

Wherever this hard sandstone is to be found outcropping hereabouts, our
forebears -
the Bronze-Age people - marked summits with large cairns.
Cairn-building, still popular today, clearly goes back a long time -
though I suspect there was a more significant purpose to the exercise
back then!
From the summit of Carn Owen, the ridge of Cerrig yr Hafan extends for
a kilometre northwards. Before it plunges into the forestry. the
remains of the Setts Quarry, a 19th Century venture that also worked
those hard Drosgol Formation sandstones, are reached:
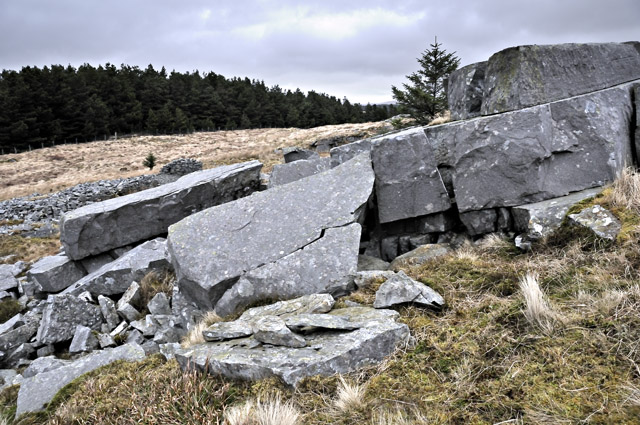
From Setts Quarry, an old tramway runs back southwards along the hill's
eastern flank, ending above two smaller quarries, separated
by
a
spoil-bank. Here's the western quarry face. The rocks can be seen to be
well-layered, and the layers are all tilted at the same angle to the
right (eastwards). The tilting is due to the strata being situated on
the east flank of an anticlinal fold - an arch-shaped structure, with
the oldest rocks in the middle and progressively younger rocks
outcropping on either side outwards.
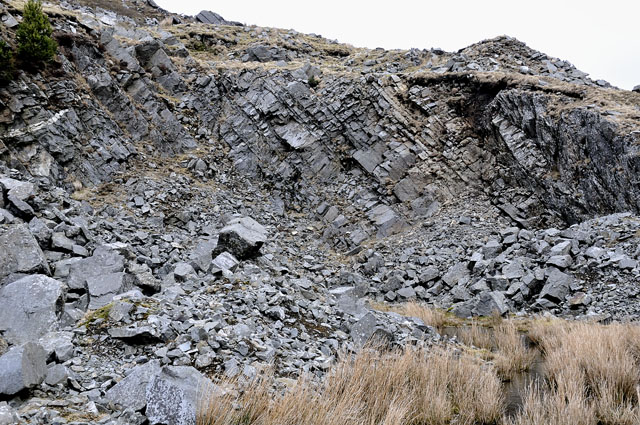
The rocks in the western quarry all belong
to the latest Ordovician, specifically the Hirnantian Stage, covering a
brief (geologically-speaking)
timespan from approximately
445.6 to 443.7 million years ago - less than a couple of million
years. They were deposited in the marine sedimentary basin that
occupied much of what is today known as Wales during the Lower
Palaeozoic era. The well-layered rocks in the image -
again hard sandstones - are the upper part of the Drosgol
Formation. To the right
of the image, relatively structureless grey rocks are present: these
belong
to the overlying Bryn-glas Formation. Let's have a closer look.
This is what the Drosgol Formation's
sandstone looks like up close.
Full of grains of quartz and feldspar, it is pale, hard and splintery.
In
some other places in the district, it also contains beds of rounded
quartz pebbles. Now, in order to transport such coarse-grained
sediments to their place of deposition, the environment needs to be one
of relatively high energy, with strong currents at work - a place of
great turbulence.

This is the eastern quarry face. Here, we are further up in the
sequence - in slightly younger rocks in other words. The grey rather
solid-looking rocks that make up the left-hand part of the exposure
belong to the top of the Bryn-glas Formation,
but
near
the
middle
a
marked
change
occurs
and they are overlain by rather
flaky, rusty-coloured rocks. The change marks the position of the
Ordovician-Silurian boundary, 443.7 million years ago. The
rusty-coloured flaky rocks of the lowermost Silurian are termed the
Cwmere Formation.

Here is a close-up of the Bryn-glas Formation - it consists of rather
monotonous massive grey silty mudstone....

...whilst here is the overlying Cwmere Formation from the Lower
Silurian. It consists primarily of dark grey mudstone which weathers to
a rusty
colour. The rusty weathering is due to the common pyrite - iron
sulphide - that the formation contains. The technical name for this
rock is "hemipelagite" - meaning deep sea mud.

Unlike the high-energy sandstones of the Drosgol Formation and the
massive mudstones of the Bryn-glas Formation, the Cwmere Formation
contains lots of fossils. This alone suggests it was deposited in a
low-energy environment - strong currents charged with sediment would
tend to smash fragile animal remains to bits.
The commonest fossils to be found in the Cwmere Formation are
graptolites (below). This specimen, with individuals 4-5cm in length,
was collected some years ago from a block of
unweathered mudstone,
from the tip of a nearby lead-mine. The
graptolites are beautifully preserved by the pyrite that has replaced
their organic
structure. Graptolites were free-floating
organisms
that existed, like modern plankton, close to the sea surface. Because
of that mode of existence, they were transported widely around on the
currents of the
ancient seas. Thus, wherever fossiliferous Lower Silurian mudstones of
marine origin are found around the world, they are likely to contain
graptolites just like these. The other important thing about
graptolites is that they evolved rapidly: appearing in the Cambrian
Period, they underwent such rapid explosions of evolution during the
Ordovician and Silurian that these two periods have been divided into
graptolite biozones - a rock's graptolite fauna can tell the geologist
which stage of which period it belongs to. Useful!
More rarely, orthocones are found in the Cwmere Formation. The one
illustrated below is an especially fine example: about 7cm long, it is
preserved in 3D by pyrite. Orthocones were cephalopods - ancient
ancestors of the modern-day Nautilus - swimming freely throughout the
seas.
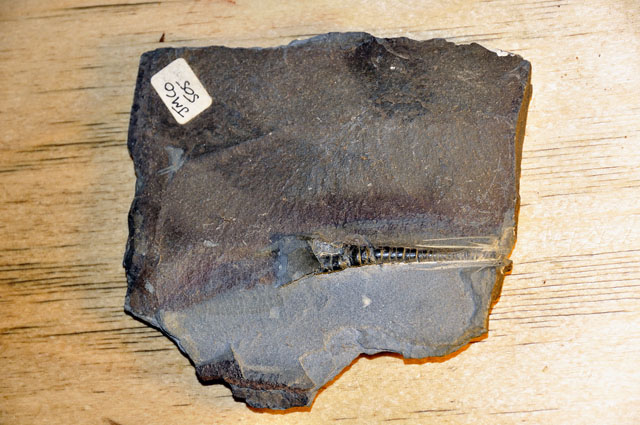
Finally, going to a microscopic scale, there are pyrite framboids
throughout the Cwmere Formation. The image below was taken down a
reflected
light microscope, looking at the surface of a chip of Cwmere Formation
mudstone that has been polished to an optical
finish. In the image, the largest
framboids - the bright-coloured spheres - are less than a millimetre
across.
What are framboids? Some
think they formed by an entirely chemical process within the sediment:
others have suggested that they represent clumps of mineralised fossil
bacteria. Either way, they record the fact that the chemical
environment on the sea-bed where the Cwmere Formation was deposited was
a strongly reducing one i.e. free oxygen was scarce or absent and the
substrate would have been stinking, sulphurous black mud.

So, in a
sequence exposed across a short distance on this mid-Wales hillside, we
have seen a transition over less than a couple of million years from
high-energy, turbulent conditions to a stagnant, deoxygenated undersea
plain where fine, muddy sediment only accumulated slowly and animal
remains were preserved in pyrite. What happened? First,
we need some context.
Palaeogeographical
reconstructions
of
the
Earth
show
how
the
continents
have
drifted around, collided and split up over the past hundreds of
millions of years. The globe below is a reconstruction of how things
would have looked at the end of the Ordovician Period, when the rocks
of Carn Owen were being deposited. England, Wales and southern Ireland
were part of a block of continental crust known as Avalonia, and to its
north lay the Iapetus Ocean, separating Avalonia from Laurentia, which
included Scotland and the north of Ireland. Avalonia lay at a low
latitude in the Southern Hemisphere and the South Pole was straddled by
the large continent of Gondwana.
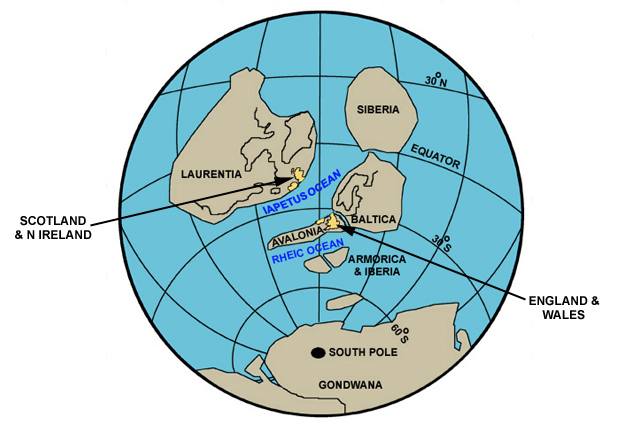
Zooming in, this is what the area that is now England and Wales would
have looked like at the time of the deposition of the Cwmere Formation.
Such maps can be created by studying the rocks: their composition
and fossil fauna and/or flora tell us about the environmental
conditions, such as sea-depth, at the time. In the early Silurian, the
Carn Owen area, just
to the north-east of where Aberystwyth is now situated, lay in a
deep marine basin on the southern margin of the Iapetus Ocean. Across
to the
east, the area that is now the Midlands was a low-lying landmass that
was partially submerged under a shallow "shelf" sea.
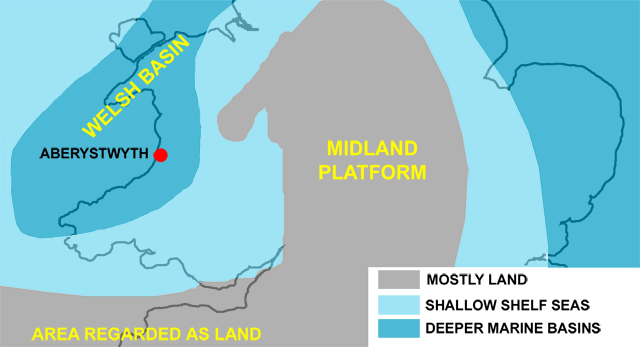
However, during the preceding Hirnantian, the map would have looked a
bit different:
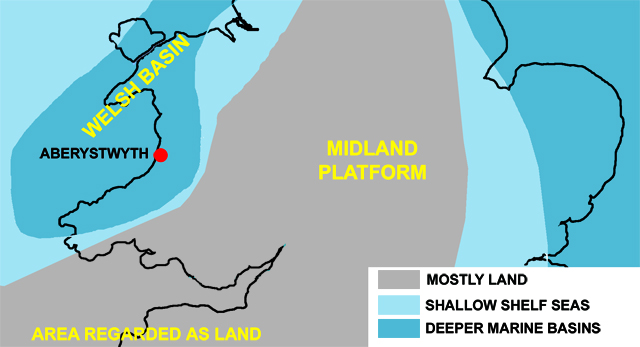
Prior to the Hirnantian, extensive shelf-seas with an abundant
bottom-dwelling fauna (indicating decent oxygen levels) covered much of
East Wales, Shropshire and Herefordshire. Then, during the Hirnantian,
sea-levels fell drastically (it has been estimated that a fall of at
least 80m was
involved - reference 3). Less than two million years later, at the
beginning of the
Silurian, the shelf-seas returned again, flooding in over the Midland
Platform.
This sudden (in geological terms) retreat and readvance of the sea had
profound effects. For a start, the retreat caused extensive areas of
shallow shelf sea, teeming with life, to become dry land. That
was one factor in the great
mass-extinction that accompanied the changes - loss of habitat.
The
land
at
the
time
was
not
densely-vegetated
like that of today: the
first primitive land-plants only appeared during the Ordovician and it
was only later in the Silurian that they really got going. As a
consequence, with nothing much holding the ground together, sediment
was readily eroded from the newly-emerged land by rainfall and rivers
alike, transported to the edge of the Welsh Basin and trundled down
into its depths by powerful submarine debris-flows, covering the basin
floor with mud, silt, abundant coarse sand and, in places, pebbles.
Thus were the Drosgol and Bryn-glas Formations deposited.
When sea-levels rose again, flooding back over the shelf, erosion of
sediments by rainfall and rivers was curtailed and the Welsh Basin
became relatively isolated from the sediment source, so that only the
finest muds settled out over its depths, onto which the remains of the
graptolites and orthocones settled out from the near-surface waters
high above. In this way, the Cwmere Formation came into being.
So what caused this sudden double-flip in sea-levels? We have to look
at the rocks that were deposited over the great southern continent,
Gondwana.
ICE-IN THE SAHARA? - GONDWANA AND THE HIRNANTIAN GLACIATION: CAUSES
Gondwana has long since split up into fragments. Bits of it now
constitute Africa,
South America, India, Antarctica and Australia. In several of these
modern continents, late Ordovician rocks occur that have features that
can only have had
one origin - from glaciers and ice-caps. Such direct indicators of
ancient glaciation that are preserved in the geological record include
things like striated rock surfaces - ice-sheets full of rocky
debris do a good job of grinding down the rocks underneath. The
striking image below shows an
example:
it illustrates a glacially-striated rock surface in Jebel Eghei, in the
Libyan Sahara Desert (photo reproduced with
thanks to Seb
Luning).
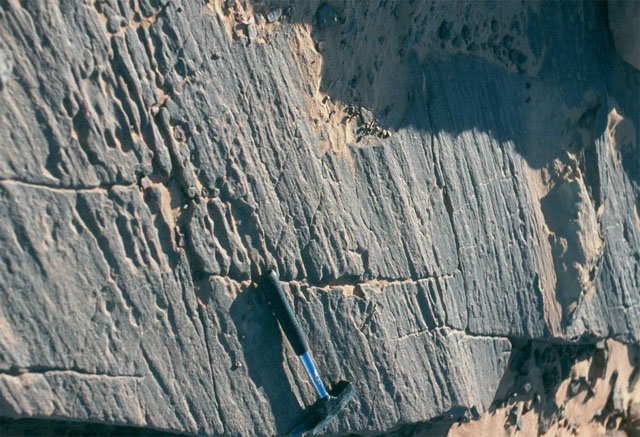
There are also indirect indicators of ancient glaciation: the
fall (and subsequent rise) in sea-levels, recorded on Carn Owen by
those
changes in rock-types described above, is to be found in marine rocks
of the same age all over the world - therefore the sea-level changes
were eustatic - global - in nature. In other words, there was a global
cooling, leading to a major ice-age, with attendant global sea-level
falls due to so much water being locked up in ice, followed by a global
warming, melting the ice and bringing worldwide sea-levels back to
"normal" again (reference 1). How, then, did it happen?
For a global cooling sufficient
to
generate
conditions
that
favour
glaciations
to
occur, as indeed also
occurred during the late Cenozoic era - the run-up to the most recent
ice-ages - there are
several variable drivers of global climate that act on the required
timescale.
The geographical arrangement of the continents
due to plate tectonics affects prevailing atmospheric and ocean
currents. The composition of
the atmosphere affects its ability to lose heat (infra-red radiation)
to space or retain it. The energy output from the sun affects the
amount of visible and ultra-violet radiation reaching Earth.
Taking geography first, having the continent of Gondwana over the South
Pole would be advantageous if you want ice-caps to start forming: it
would have played a role similar to that of Antarctica today. So that's
one box ticked.
As to
atmospheric composition, it appears that carbon dioxide levels
during the Ordovician were much higher than present atmospheric levels,
running at several thousand parts per million (ppm). Palaeotemperature
data suggest that, through
much
of
the
Ordovician,
Earth
had
a
stable
Hothouse-type
climate
similar to that during the late Mesozoic and the early Cenozoic.
It seems that a fairly stable carbon-cycle
existed, with a balance between sources such as volcanic outgassing and
sinks such as ocean uptake.
However, in the late Ordovician, this relative stability seems to have
been disturbed. Major episodes of mountain-building and volcanic
activity occurred only a few
million
years prior to the Hirnantian glaciation, such as the Taconic Orogeny,
which affected what is now eastern North America. When
mountain-building occurs, erosion increases by orders of magnitude as
the newly-uplifted ranges develop drainage-systems: the erosion
massively increases the surface area of material available to
weathering agents like rain - carrying dissolved carbon dioxide - and
other
substances. Weathering of rocks is thus a major carbon-sink, so that
any mountain-building episode has the potential to create a
significant new carbon dioxide drawdown.
Geochemical
models
track fluxes of carbon entering and exiting the atmosphere. One
such well-regarded model, which predicts carbon dioxide levels for the
entire
Phanerozoic, is GEOCARB, with a time-step of 10 million years
(reference 2). However, this is too coarse a resolution to pick up any
shortlived major dip in carbon dioxide levels around the
geologically brief cold period of the Hirnantian
(reference 6). Can
we
find
good
evidence
of
enhanced
carbon
dioxide
drawdown
at this time, via
other methods?
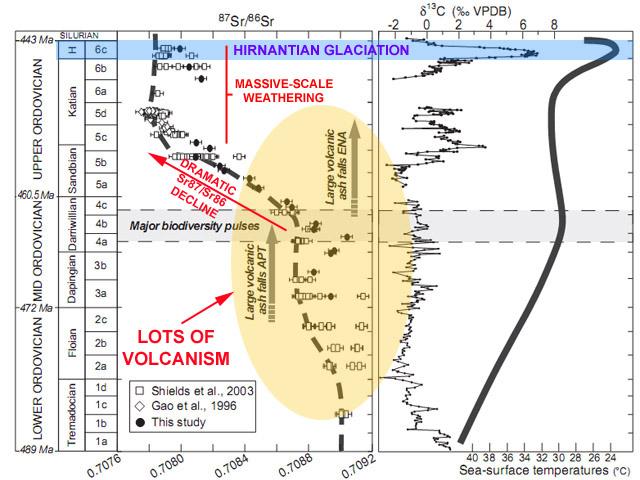
It appears so. Recent research (diagram above - with my colour
annotations - from reference 9) has looked
at the ratios
of strontium
isotopes in post-Taconic (late Ordovician) limestones in North America.
The later Ordovician limestones showed a dramatic Sr87/Sr86 ratio
decline to much lower values (LH plot, above): indeed, the paper's
abstract says: "A large drop in
seawater 87Sr/86Sr during the Middle Ordovician was among the most
rapid in the entire Phanerozoic." The research indicated that
this trend could well be due to the onset of massive-scale weathering
of basalts - igneous rocks enriched in
strontium-86. Basalts consist mainly of various silicates of calcium,
sodium,
magnesium and iron, all of which readily react with carbon dioxide
dissolved in
rainwater,
the resultant solutions reprecipitating the calcium and carbonate ions
as limestone. In addition to the effect of this carbon-sink, the
widespread volcanism of the Middle Ordovician was on the wane, reducing
carbon dioxide
outgassing. More specifically, the abstract of Reference 9 goes on to
state:
"A close balance between volcanic outgassing and CO2 consumption from
weathering produced steady pCO2 levels and climate through the middle
Katian, consistent with recent Ordovician paleotemperature estimates.
In the late Katian, outgassing was reduced while volcanic weathering
continued, and resulted in a cooling episode leading into the
well-known end-Ordovician glaciation."
So yes, it appears that carbon dioxide levels did drop
quite sharply
for a time in the late Ordovician: indeed, it has been estimated that
they fell from 5000ppm to 3000ppm or less (reference 6) as a
consequence of the events described.
3000ppm of
carbon dioxide
still sounds high, but before jumping to conclusions popular elsewhere
in the blogosphere, we still have another main driver to take into
account. What about
the Sun? Established nuclear models of main sequence stars indicate
that it would have been 4-5% dimmer than it is today (references 5,6).
Solar radiance is thought to have increased in a pretty much linear
fashion by about 10% per billion
years of Earth's history and the following graph charts this increase
through the Phanerozoic:

What difference would 4-5% less incoming solar energy make? Global
climate models, calibrated to
today's conditions, predict that permanent ice can start to develop
when carbon dioxide
levels drop below 500 ppm (i.e. if all of Earth's icecaps melted in the
future due to global warming caused by very high, say 800ppm, carbon
dioxide levels, they would eventually start to reform if those levels
again fell below the 500 ppm mark). Calibrating the
models to the mid-Cenozoic gives a range for this "ice threshold" of
560–1120 ppm carbon dioxide, and as
Royer (reference 6) notes,
global climate models and energy balance models
calibrated to Late Ordovician conditions, with 4-5% less solar
irradience, predict an ice
threshold of between 2240 and 3920 ppm carbon dioxide. So: combine a
dimmer sun, a
sudden drop in carbon dioxide levels to within the ice-threshold area
and a large continent over the South Pole and
that appears to have been sufficient to get the temperature low enough
to allow glaciation to develop, as the consequence of several key
climate drivers working in conjunction with one another.
Was there an alternation of glacials and interglacials, as in the
recent Pleistocene events? It appears so: deposits in what was North
Gondwana record two well-defined ice-related depositional sequences
separated by major erosional unconformities (reference 1). The
Milankovitch (orbital) forcings were not necessarily just restricted to
the last Ice Age's sequence of alternately advancing and retreating
ice-sheets.
How did temperatures and carbon dioxide levels bounce back into the
early Silurian? This remains problematic (reference 1): weathering of
carbonate sediments on the uncovered shelf-sea areas in the relative
absence of calcifying plankton (i.e. mass-extinction had partially
disabled an important carbon-sink) may have been one reason; strongly
reduced silicate weathering-rates in glaciated areas has also been
suggested: either would increase atmospheric carbon dioxide levels
again. Like many such things of the dim and distant past, there remains
plenty of scope for worthwhile research!
THE LATE ORDOVICIAN MASS-EXTINCTION
According to the fossil record, during the late Ordovician glaciation,
26% of all
families and 60% of all genera of both terrestrial and marine life
worldwide were exterminated (reference
8). It was the
second-biggest such event in the fossil record - the huge end-Permian
extinction being the most spectacular, as the sketch-graph below shows.

We know that
the extinction accompanied a period of rapid climate change that
followed, and was followed by, periods of high mean global
temperatures. The extinction took place over a couple of million years
- as
opposed to relatively sudden disaster-movie events like the K-T
boundary
extinction: indeed, it appears to reflect a prolonged period of
environmental stresses, including the aforementioned major fluctuations
in sea level associated with glaciation and accompanying changes to
ocean temperatures and chemistry. Oceanic changes are particularly
implicated in
the loss of coral-reefs: although some individual species of corals
survived, living reefs themselves disappeared from the face of the
Earth. Although the mass-extinction was followed by a Lower Silurian
biodiversity "explosion", it took as much as 6 million years for coral
reefs to reappear,
making the episode the first true ‘reef gap’ in the geological record.
The detailed explanation for these stresses is outside the scope of
this post (see references 7 and 8 for further reading) and is indeed a
subject of ongoing research. However, the important point should be
made that any major and geologically rapid climate shift in either
direction - warmer or cooler - from a stable state brings with it
drastic environmental changes that can have profound effects on
ecosystems and create the potential for extinction-level
events. It should also be pointed out that our burning of the fossil
fuels on such a massive scale over just a couple of centuries puts the
current environmental and atmospheric changes up there with the bigger
examples of the past.
It's surprising what the geology of a couple of small, grey crags on a
Mid-Wales hillside can lead one into. Interestingly, across the
Nant-y-Moch reservoir, on the shoulder of the hill called Drosgol
(after which the Formation was named), there is evidence of another
period of Earth's climate, where rocks and minerals along a fault-line
show evidence of such pervasive, penetrating chemical weathering that
they likely record another bygone age. This time, they point to the
Cenozoic era, when Wales had a tropical climate, before the long-term
cooling that led to the more recent glaciations that shaped the modern
Welsh landscape. Something for another post, possibly: another excuse
for an afternoon tramping these hills, with their hidden geological
surprises!
REFERENCES:
Internet:
Geological Timescale - Subcommission
for Stratigraphic Information
Publications (Google for PDFs in most cases):
1) Armstrong,
H.A. (2007): On the cause of the Ordovician glaciation. In:
Williams, M., Haywood, A.M., Gregory, J. and Schmidt, D.N. (eds):
Deep-time perspectives on climate change: marrying the signal from
computer models and biological proxies, 101-123. Published by the
Geological Society for the Micropalaeontological Society. ISBN:
1-86239-240-4.
2)
Berner,
R.A.
and
Kothavala,
Z.
(2001):
GEOCARB
III:
a
revised
model
of atmospheric CO2 over Phanerozoic time.
3) Brenchley,
P.J., Marshall, J.B, Harper, D.T., Buttler, C.J. and
Underwood, C.J. (2006): A late Ordovician (Hirnantian) karstic surface
in a submarine channel, recording glacio-eustatic sea-level changes:
Meifod, central Wales. Geological Journal 41: 1–22.
4) Cave, R. and Hains, B.A. (1986): Geology of the country between
Aberystwyth and Machynlleth . Memoir of the British Geological Survey,
Sheet 163 (England and Wales).
5) Minton, D.A. and Malhotra, R. (2007): Assessing the massive young
sun
hypothesis to solve the warm young earth puzzle. The Astrophysical
Journal, 660: 1700-1706.
6) Royer, D.L.
(2006): CO2-forced climate thresholds during the
Phanerozoic. Geochimica et Cosmochimica Acta 70, 5665–5675.
7) Sandberg, P.A. (1983): An oscillating trend in Phanerozoic
non-skeletal carbonate mineralogy. Nature 305: 19–22.
8) Veron, J.E.N. (2008): Mass extinctions and ocean acidification:
biological constraints on geological dilemmas. Coral Reefs 27, 459–472.
9) Young, S.A., Saltzman, M.R., Foland, K.A., Linder, J.S. and Kump,
L.R. (2009): A major drop in seawater 87Sr/86Sr during the Middle
Ordovician (Darriwilian): Links to volcanism and climate? Geology,
October 2009, v. 37, p. 951-954.
|
BACK TO WEATHER-BLOG MENU
New!
Fine
Art
Prints
&
digital
images
for
sale-
Welsh Weather & Dyfi Valley landscapes Slide-Library - Click HERE |
|
|